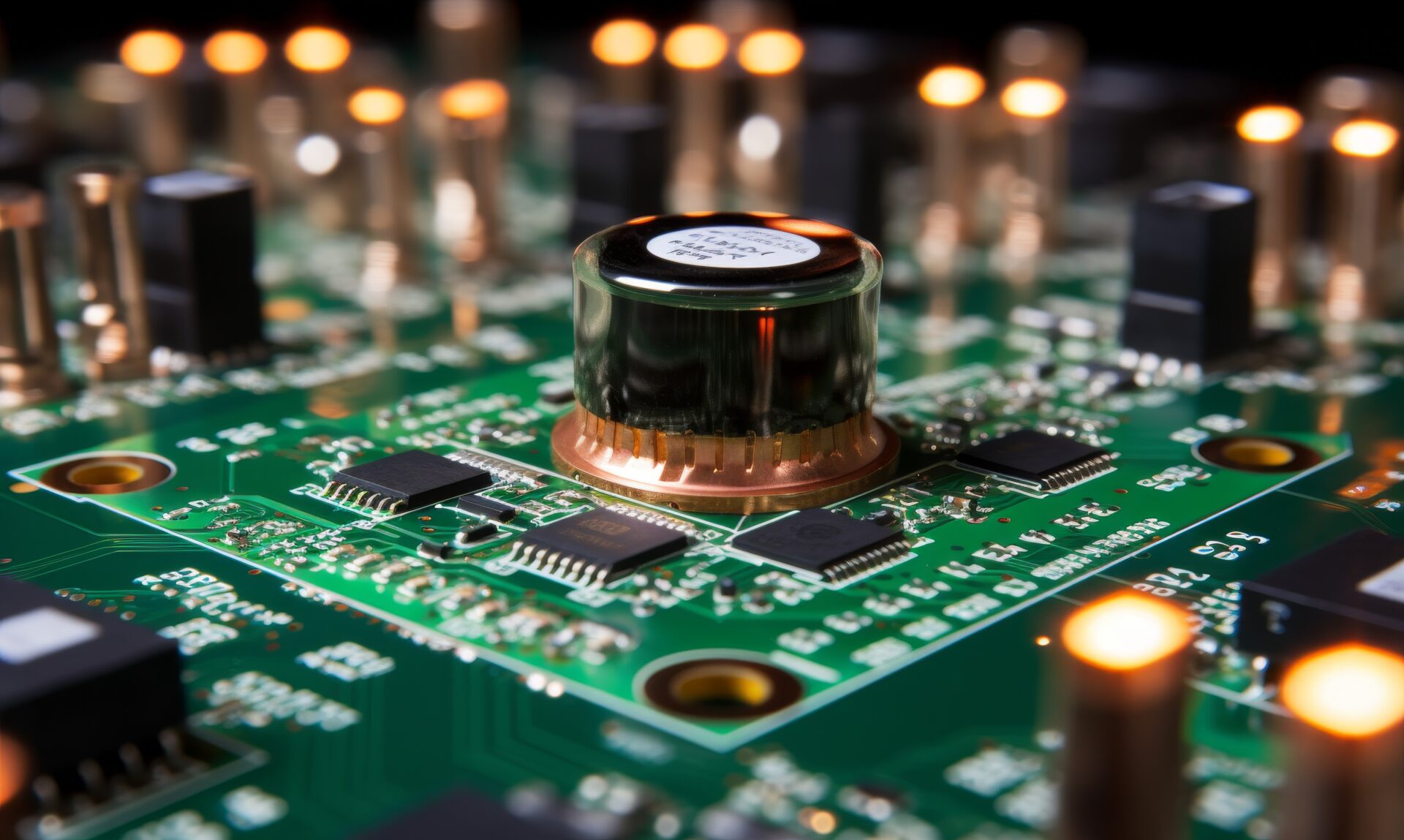
- Photonics
Three Types of Photodiodes and Their Structures and Operating Principles
Contents
Three types of photodiodes and how to choose between them
Photodiodes are semiconductor devices that convert light into electrical signals. They have various uses where photoelectric conversion is required such as sensors that react to light or measure light intensity, laser pickups for optical discs such as DVDs, and optical signal detection in optical communications. There are three main types of photodiodes, with differing structures and operating principles: PN photodiodes, PIN photodiodes, and avalanche photodiodes (APDs). In this article, we will discuss the structures and characteristics of each.
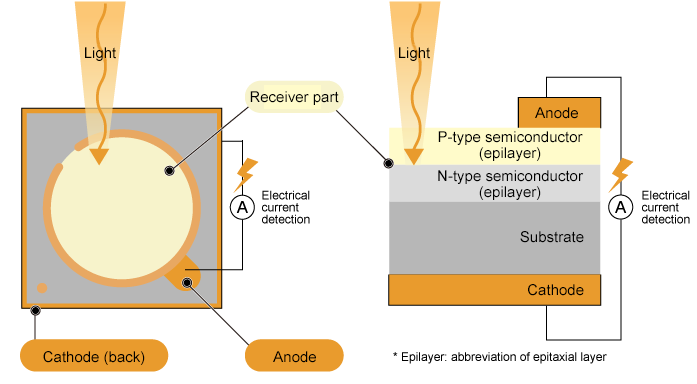
We will begin with three key terms that often come up when discussing photodiodes: photosensitivity, dark current (noise), and response speed.
Photosensitivity indicates how efficiently a photodiode can absorb light to generate electrons and holes. The higher the photosensitivity, the greater the electric current produced.
Dark current is a small electric current that runs through a photodiode even when no light is present. It is caused by naturally occurring carriers (electrons and holes) due to external heat as well as excitation of electrons due to crystal defects and impurities. It is necessary to suppress dark current generation as much as possible since it creates sensor noise that reduces measurement precision and sensitivity.
Response speed is often represented as the time interval from the point at which light enters a photodiode until electric current is generated and flows out. Various factors affect response speed, such as the thickness of the depletion layer within the device, the magnitude of the reverse bias voltage, device area, and operating temperature. As these factors change the speed at which electrons travel inside the semiconductor, response speed changes as well.
PN photodiodes, PIN photodiodes, and avalanche photodiodes (APDs) differ in their characteristics, making it necessary to select a type based on the application.
The structure and operating principles of PN photodiodes
Below, we will discuss the structures and operating principles of each type of photodiode.
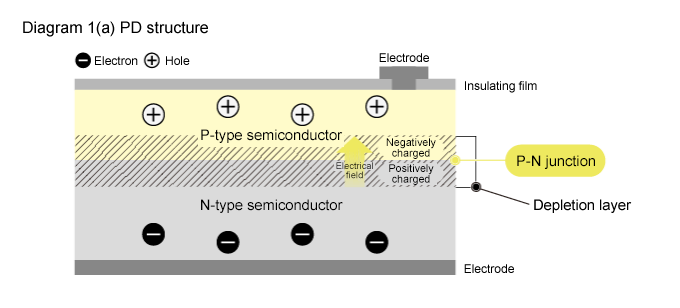
PN photodiodes are photodiodes with a basic structure. They consist of a p-type semiconductor and n-type semiconductor joined together with electrodes at both ends, similar to a photovoltaic cell.
PN photodiodes work as follows: when two different types of semiconductors are joined to form a PN semiconductor, excess electrons move from the n-type semiconductor to the p-type semiconductor to join with holes. As a result, an area called the depletion layer is formed near the PN junction. In the depletion layer, the n-type side takes on a positive charge and the p-type side takes on a negative charge. The charge imbalance in the depletion layer generates an internal electric field. This electric field makes it possible for the PN photodiode to operate without applying external voltage.
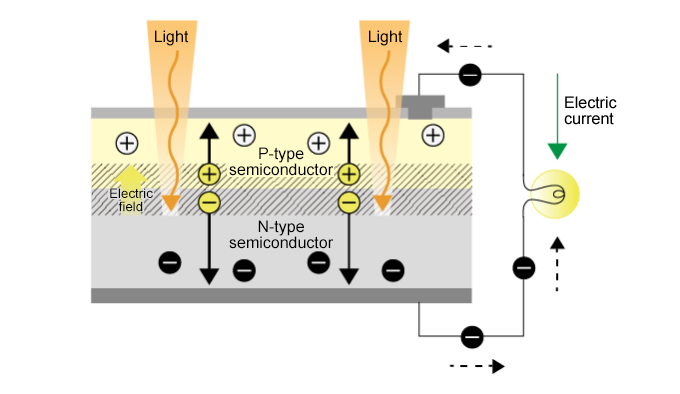
As seen in the diagram above, when light enters the depletion layer of a PN photodiode, the light energy creates electron-hole pairs. Due to the internal electric field, electrons move toward the electrode on the n-type semiconductor side and holes move toward the electrode on the p-type semiconductor side. This flow of electrons and holes continues as long as light is applied. The simple structure of PN photodiodes gives them the advantages of ease of manufacturing, low cost, and stable operation. While response speed is slow, dark current is low. As a result, they are widely used in light intensity measurement.
The structure and operating principles of PIN photodiodes
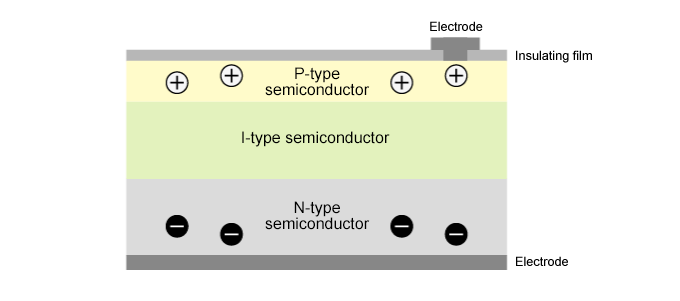
PIN photodiodes are light detection devices with a structure that is improved over conventional PN photodiodes. As the name indicates, the structure includes an intrinsic (i-type) layer of pure semiconductor between the p-type semiconductor layer and n-type semiconductor layer. This design achieves fast response speed and high sensitivity while maintaining low dark current.
In conventional PN photodiodes, light is primarily absorbed in the narrow depletion layer that naturally forms near the junction of the p-type layer and n-type layer. In PIN photodiodes, on the other hand, the wide i-type layer is deliberately introduced to fulfill this role. This i-type layer consists of a pure semiconductor with few electrons or holes, allowing it to absorb light efficiently.
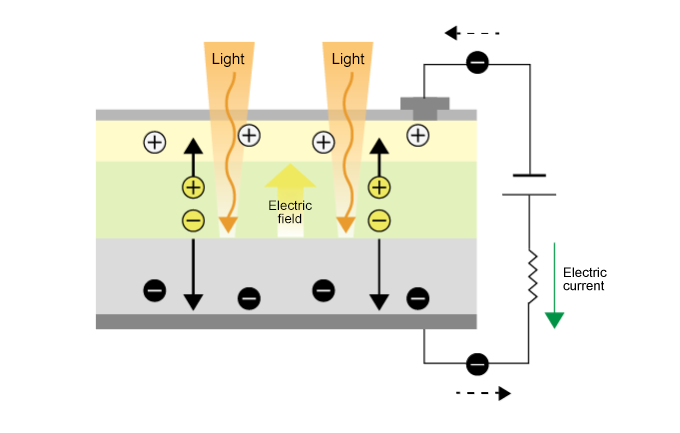
While a PIN photodiode is operating, reverse bias voltage is applied to the i-type layer. This forms a strong electric field within the i-type layer. When light hits the i-type layer, photon energy creates electron-hole pairs. The generated electrons and holes move at high speed due to the influence of the strong electric field. Specifically, electrons move toward the n-type layer’s electrode and holes move toward the p-type layer’s electrode. This high-speed movement creates a strong electric current.
The main advantages of PIN photodiodes are high response speed, high sensitivity, and low dark current. The high response speed is a result of high-speed carrier movement due to the strong electric field, and the high sensitivity is a result of efficient light absorption in the thick i-type layer. The i-type layer’s low carrier concentration contributes to low dark current as well.
Thanks to these characteristics, PIN photodiodes are widely used in a variety of high-performance applications. In particular, they play critical roles in optical communication receivers that require high-speed data transmission, medical imaging devices that require detection of weak signals, and more.
The structure and operating principles of avalanche photodiodes (APDs)
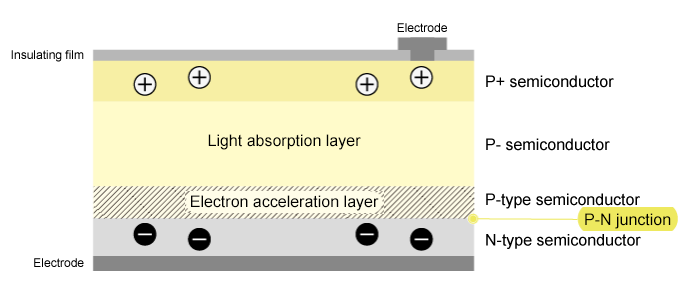
Avalanche photodiodes (APDs) are high-performance light detection devices with significantly higher performance than conventional photodiodes. Their key characteristic is high sensitivity due to the internal photoelectron multiplication effect. This makes them especially well-suited to detecting weak light signals.
APD structures are more complex than standard PN or PIN photodiodes, featuring a multilayered structure primarily composed of p-type semiconductors. Specifically, there are three layers (p+, p-, and p) with each playing its own role. The p+ layer has high carrier concentration, while the p- layer has relatively low carrier concentration. These structural characteristics enable theunique operation of APDs.
The APD’s operating principle is based on a phenomenon known as avalanche multiplication. The process is as follows:
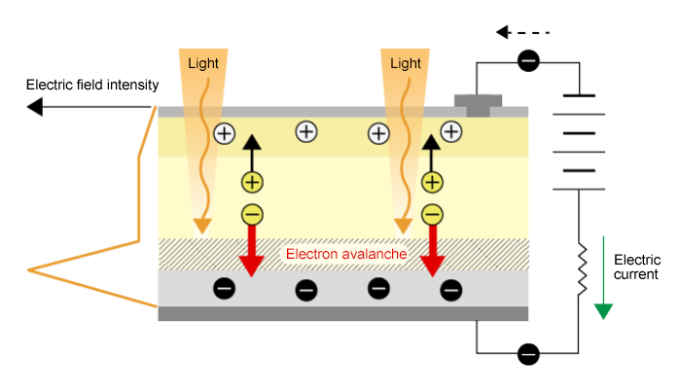
- Light absorption: the p- layer absorbs light and generates electron-hole pairs.
- Electron acceleration: the generated electrons are accelerated by a strong electric field toward the p-type layer.
- Electron collision: accelerated electrons collide with atoms in the semiconductor and generate new electron-hole pairs.
- Chain-reaction multiplication: the newly generated electrons are also accelerated, causing further collisions and electron-hole pair generation.
- Electron avalanche: this chain reaction leads to an avalanche-like increase in the number of electrons and holes.
This electron avalanche achieves extremely high sensitivity for APDs. Even weak light signals are significantly amplified to create strong electric currents that can be detected.
APDs require high voltage to operate. The electric fields in each layer are not uniform, and making the p-type layer thin creates a large electric field gradient in the depletion layer. This strong electric field gradient enables efficient electron avalanches.
Due to their excellent performance, APDs play important roles in a wide range of fields such as optical communication receivers, astronomical observation in low-light conditions, and scientific instruments that require high sensitivity. In particular, they truly shine when the detection of weak light signals is required.
There are also challenges when using APDs, however. The complex structure and high-voltage operation requirement lead to high manufacturing costs, making them more expensive than other types of photodiodes. In addition, expert handling such as temperature control and precise voltage control are necessary for proper operation.
While APDs can deliver high performance, they require deep expertise and an appropriate operation environment. Despite this, their exceptional sensitivity is indispensable in many cutting-edge applications, contributing to the development of optical engineering technology.
- SHARE